WHAT IS THE DIFFERENCE BETWEEN RAIN AND PRECIPITATION?
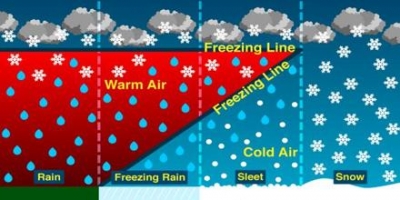
When a lot of water vapour fills the air, it begins to change and condense into droplets of water. These droplets fall back onto Earth as precipitation, which can take many forms - rain, hail, snow, sleet, fog, dew. So rain is just one form of precipitation. |
The difference between Rainfall and Precipitation is that the Rainfall is a liquid water in the form of droplets that have condensed from atmospheric water vapor and then precipitated and Precipitation is a product of the condensation of atmospheric water vapour that falls under gravity.
Rainfall
Rain is liquid water in the form of droplets that have condensed from atmospheric water vapor and then becomes heavy enough to fall under gravity. Rain is a major component of the water cycle and is responsible for depositing most of the fresh water on the Earth. It provides suitable conditions for many types of ecosystems, as well as water for hydroelectric power plants and crop irrigation.
The major cause of rain production is moisture moving along three-dimensional zones of temperature and moisture contrasts known as weather fronts. If enough moisture and upward motion is present, precipitation falls from convective clouds (those with strong upward vertical motion) such as cumulonimbus (thunder clouds) which can organize into narrow rainbands. In mountainous areas, heavy precipitation is possible where upslope flow is maximized within windward sides of the terrain at elevation which forces moist air to condense and fall out as rainfall along the sides of mountains. On the leeward side of mountains, desert climates can exist due to the dry air caused by downslope flow which causes heating and drying of the air mass. The movement of the monsoon trough, or intertropical convergence zone, brings rainy seasons to savannah climes.
The urban heat island effect leads to increased rainfall, both in amounts and intensity, downwind of cities. Global warming is also causing changes in the precipitation pattern globally, including wetter conditions across eastern North America and drier conditions in the tropics. Antarctica is the driest continent. The globally averaged annual precipitation over land is 715 mm (28.1 in), but over the whole Earth it is much higher at 990 mm (39 in). Climate classification systems such as the Köppen classification system use average annual rainfall to help differentiate between differing climate regimes. Rainfall is measured using rain gauges. Rainfall amounts can be estimated by weather radar.
Rain is also known or suspected on other planets, where it may be composed of methane, neon, sulfuric acid, or even iron rather than water.
Precipitation
In meteorology, precipitation is any product of the condensation of atmospheric water vapor that falls under gravity. The main forms of precipitation include drizzle, rain, sleet, snow, graupel and hail. Precipitation occurs when a portion of the atmosphere becomes saturated with water vapor, so that the water condenses and “precipitates”. Thus, fog and mist are not precipitation but suspensions, because the water vapor does not condense sufficiently to precipitate. Two processes, possibly acting together, can lead to air becoming saturated: cooling the air or adding water vapor to the air. Precipitation forms as smaller droplets coalesce via collision with other rain drops or ice crystals within a cloud. Short, intense periods of rain in scattered locations are called “showers.”
Moisture that is lifted or otherwise forced to rise over a layer of sub-freezing air at the surface may be condensed into clouds and rain. This process is typically active when freezing rain occurs. A stationary front is often present near the area of freezing rain and serves as the foci for forcing and rising air. Provided necessary and sufficient atmospheric moisture content, the moisture within the rising air will condense into clouds, namely stratus and cumulonimbus. Eventually, the cloud droplets will grow large enough to form raindrops and descend toward the Earth where they will freeze on contact with exposed objects. Where relatively warm water bodies are present, for example due to water evaporation from lakes, lake-effect snowfall becomes a concern downwind of the warm lakes within the cold cyclonic flow around the backside of extra tropical cyclones. Lake-effect snowfall can be locally heavy. Thunder snow is possible within a cyclone’s comma head and within lake effect precipitation bands. In mountainous areas, heavy precipitation is possible where upslope flow is maximized within windward sides of the terrain at elevation. On the leeward side of mountains, desert climates can exist due to the dry air caused by compressional heating. Most precipitation occurs within the tropics and is caused by convection. The movement of the monsoon trough, or inter-tropical convergence zone, brings rainy seasons to savannah climes.
Precipitation is a major component of the water cycle, and is responsible for depositing the fresh water on the planet. Approximately 505,000 cubic kilometres (121,000 cu mi) of waterfalls as precipitation each year; 398,000 cubic kilometres (95,000 cu mi) of it over the oceans and 107,000 cubic kilometres (26,000 cu mi) over land. Given the Earth’s surface area, that means the globally averaged annual precipitation is 990 millimetres (39 in), but over land it is only 715 millimetres (28.1 in). Climate classification systems such as the Köppen climate classification system use average annual rainfall to help differentiate between differing climate regimes.
Precipitation may occur on other celestial bodies, e.g. when it gets cold, Mars has precipitation which most likely takes the form of frost, rather than rain or snow.
Credit: maindifference.net
Picture credit: Google